We are an experimental research group in condensed matter physics and chemical physics. Using the uniquely designed scanning probe microscopes[see Nat Rev Methods Primers 1, 36 (2021)], we investigate the electronic, vibrational, magnetic, and optical properties of single molecules and low-dimensional materials, as well as the related ultrafast dynamics processes. The on-going research directions include:
1. H-bonding structure and dynamics of interfacial water
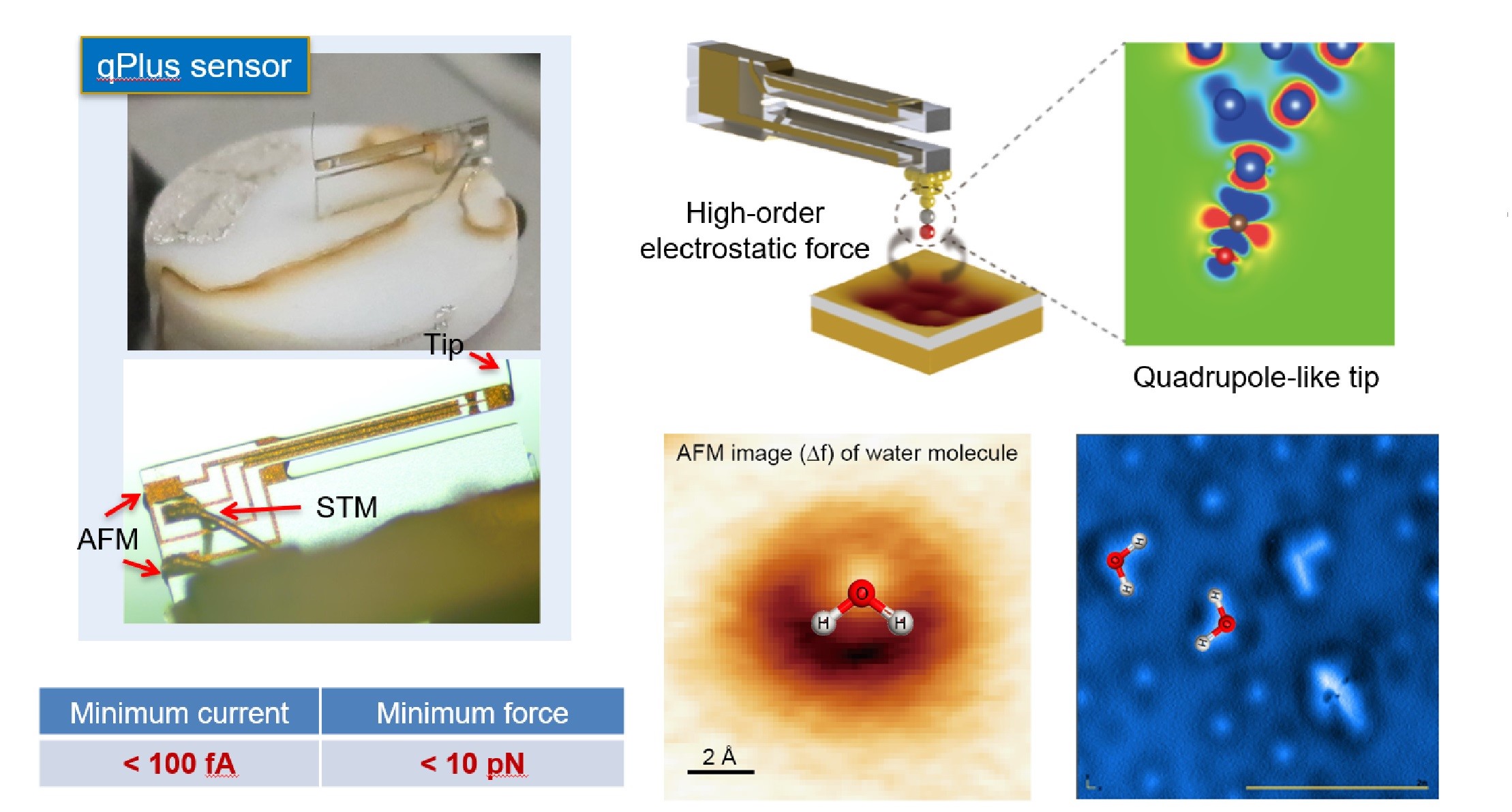
Interfacial water is ubiquitous in nature and plays an essential role in a broad spectrum of physics, chemistry, biology, energy and materials science. Many remarkable physical and chemical properties of water are governed by H-bonding interaction between water molecules. Our group is focused on achieving the atomic-scale description of H-bonding structure and dynamics using scanning probe techniques, which may help to solve the mystery of water. Based on a qPlus sensor, we have developed a new-generation scanning probe microscopy/spectroscopy with ultrahigh sensitivity and resolution. On the one hand, we are able to achieve the submolecular resolution of water molecules[1], which allows locating, in real space, the position of H atoms within the H-bonded network; On the other hand, the disturbance of the probe on the fragile water structure was reduced to a nearly non-invasive level[2]. Those innovations in SPM techniques provide us unprecedented opportunity to identify the topology of H-bonding networks[3], track the proton dynamics[4], unravel the quantum nature of H bond[5], probe the water-ion interaction[6], and image the ice growth at atomic scale[3].
[1] J. Guo. et al., Nature Materials 13, 184-189 (2014).
[2] J. Peng. et al., Nature Communications 9, 122 (2018).
[3] R. Ma. et al., Nature 577, 60 (2020).
[4] X. Meng. et al., Nature Physics 11, 235-239 (2015).
[5] J. Guo. et al., Science 352, 321-325 (2016).
[6] J. Peng. et al., Nature 557, 701 (2018).
2. Atomic-scale ultrafast dynamics
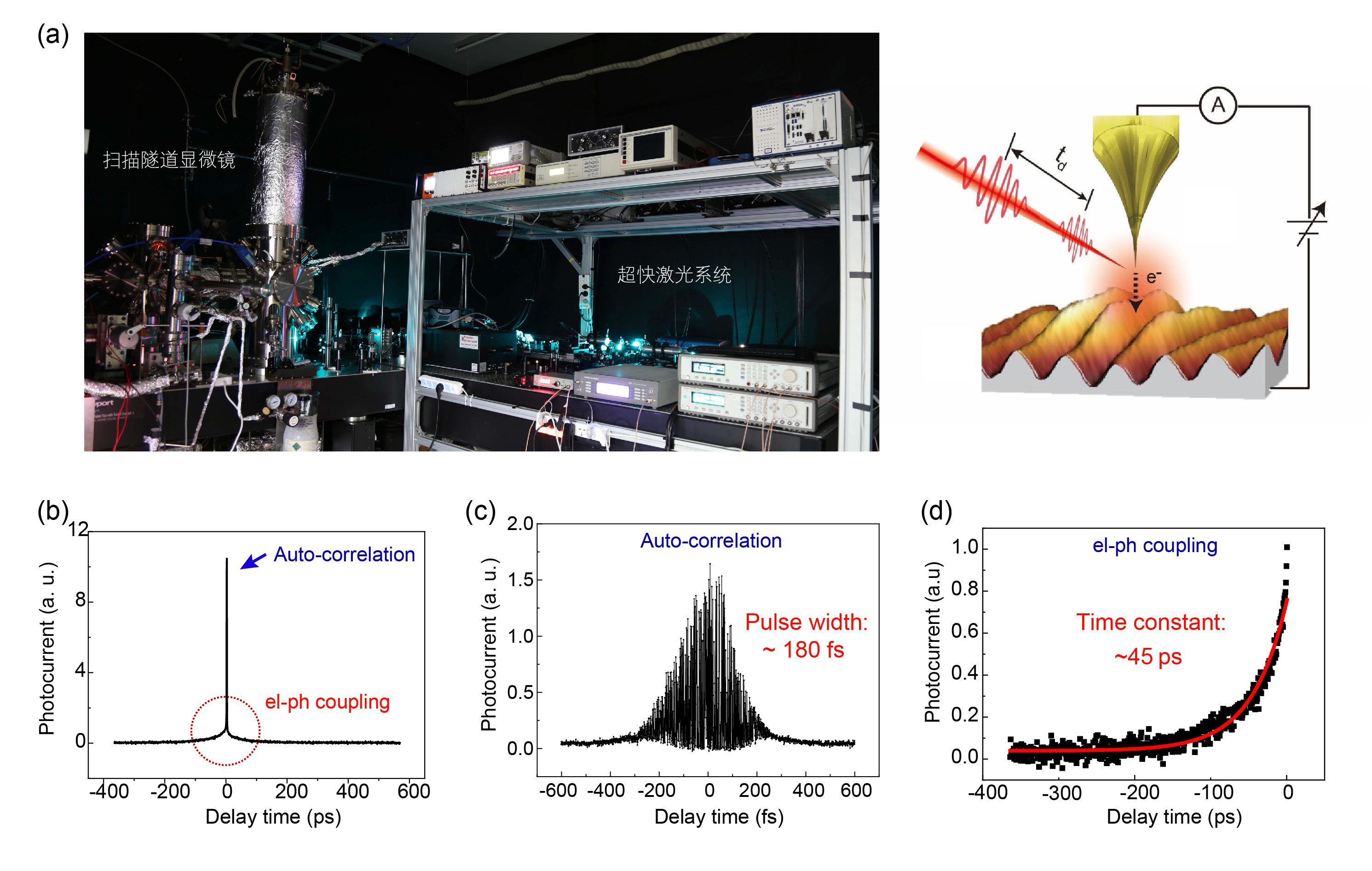
Making smaller and faster functional devices has led to an increasing demand for a microscopic technique that allows the investigation of carrier and phonon dynamics with both high spatial and temporal resolutions. Traditional optical pump–probe methods can achieve femtosecond temporal resolution but fall short in the spatial resolution due to the diffraction limit. Scanning tunneling microscopy (STM), on the contrary, has realized atomic-scale spatial resolution relying on the high sensitivity of the tunneling current to the tip-sample distance. However, limited by the electronics bandwidth, STM can only push the temporal resolution to the microseconds scale, restricting its applications to probe various ultrafast dynamic processes. The combination of these two methods takes advantages of optical pump–probe techniques and highly localized tunneling currents of STM, providing one viable solution to track ultrafast dynamics with atomic precision [1-2]. To this end, we have designed and constructed an ultrahigh-vacuum low-temperature (5 K) laser-combined STM system with excellent thermal stability (thermal drift < 0.1 pm/min), which can achieve 100-fs temporal resolution and atomic resolution simultaneously. With this state-of-the-art technology, we aim at probing various ultrafast dynamics in single molecules and low-dimensional materials [3-4], such as photocatalysis, photoelectric conversion, spin/charge dynamics, electron-phonon coupling, etc.
[1] Y. Terada. et al., Nature photonics 4, 869 (2010).
[2] Y. Tian. et al., Surface Review and Letters 25, 1841003 (2018).
[3] C. Guo. et al., Chinese Physics B 27, 077301 (2018).
[4] C. Guo. et al., Physical Review Letters 124, 206801 (2020).
3. Nanoscale quantum sensing
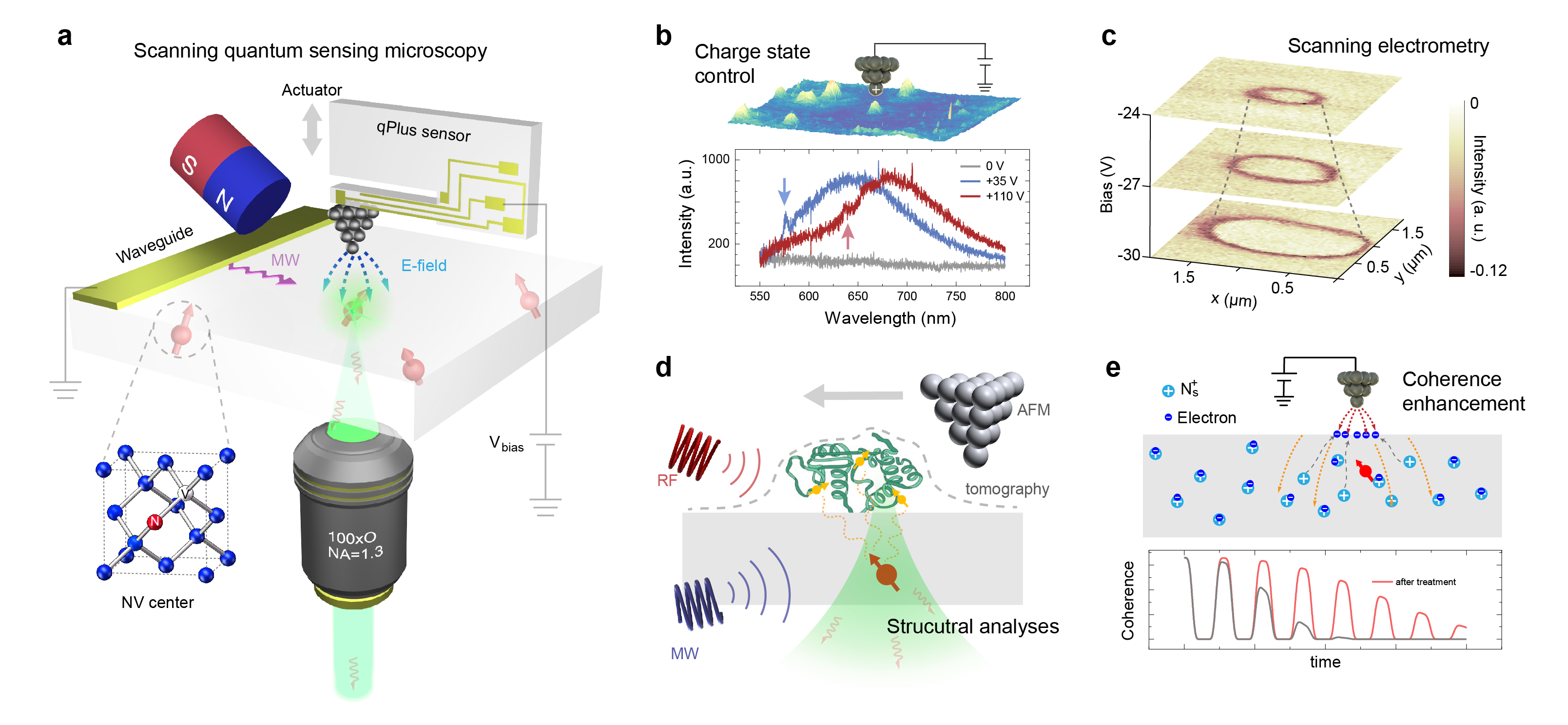
Recently, nitrogen-vacancy center (NV) host in diamond has been viewed as a wonderful quantum sensor, with high sensitivity based on long-range dipolar interaction. With the help of long coherence time and advanced spin manipulation techniques, NV is able to sense subtle electric and magnetic signals relying on phase accumulation of superposition states1 [1]. Such powerful quantum probes surpassed the sensitivity of classic measurements and succeeded in detecting single elementary charge, single electron spin and single nuclear spin with atomic resolution even under ambient conditions.1-3. Furthermore, state-of-art quantum sensing scheme have realized detection of nanoscale chemical shift, which is important for high resolution structural analyses [2]. We have built up a new type of NV-SPM, which combines both high spatial resolution with the ultrasensitive NV-based quantum sensing technique [3]. Such a system is suitable for a wide spectrum of fields such as structural analyses of complex biomolecules, in-situ chemical identification at nanoscale, structural and dynamical investigation under buried interfaces and microscopic research for ion transport in the confined geometry, etc. Our system is suitable for scanning electrometry and charge state control by using the conductive tip as a powerful local gate [4]. With such strong local field, we can engineer the NV’s immediate electrostatic environment and then pave the way for both improving the coherence, or sensitivity of shallow NV centers and constructing quantum networks or quantum processors with high spatial resolution.
[1] J. Taylor. et al., Nature Physics 4, 810-816 (2008).
[2] N. Aslam. et al., Science 357, 67-71 (2017).
[3] K. Bian. et al., Nature Communications 12, 2457 (2021).
4. Light-element quantum materials
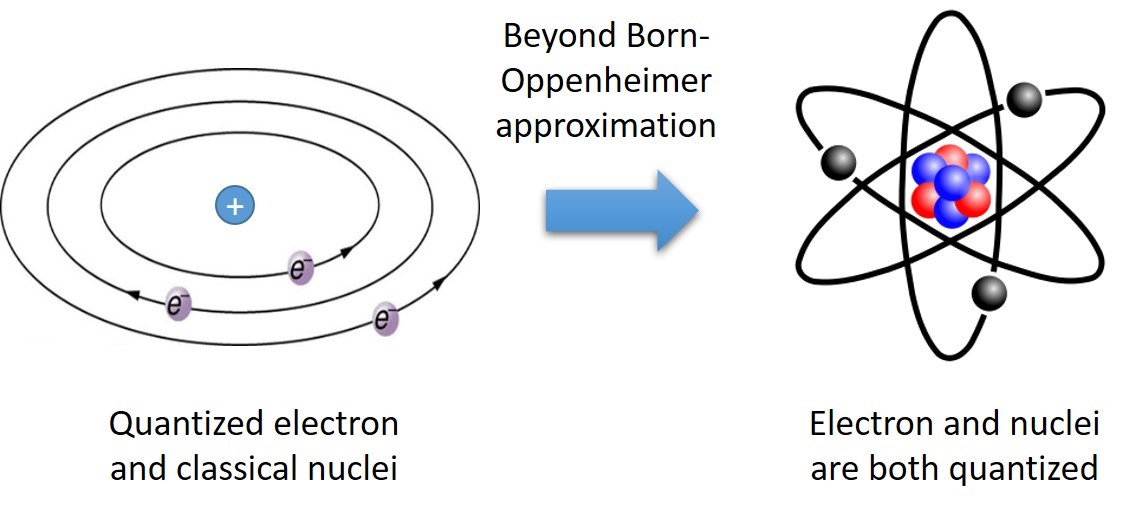
In 1926, Schrödinger proposed an equation to describe the quantum behavior of microscopic particles. Unfortunately, it is impossible to solve this equation for realistic material systems due to the many-body problem. One year later, Born and Oppenheimer avoided this difficulty by adding an approximation that only the electrons are treated quantum mechanically while the heavy nuclei are considered as classical particles. The so-called Born-Oppenheimer approximation is very successful in understanding and predicting physical properties of many materials. However, it is not accurate anymore or even invalid for light-element materials, where the nuclei can also exhibit prominent quantum effects (zero-point motion and tunneling). H-rich materials like water are probably the most noticeable archetype of such materials beyond the Born-Oppenheimer approximation due to the small mass of hydrogen nuclei [1]. In addition to H, the nuclear quantum effects of other light elements, such as He, B and C, can also play an important role. Those light-element materials can display exotic quantum properties due to the quantization of both electron and nuclei, such as high-temperature superconductivity [2], supersolidity [3], and abnormalous isotopic effects [4].
[1] M. Ceriotti. et al., Chemical reviews 116, 7529-7550 (2016).
[2] F. Errea. et al., Nature 578, 66-69 (2020).
[3] E. Kim. et al., Nature 427, 225-227 (2004).
[4] T. Vuong. et al., Nature Material 17, 152 (2018).